Researchers in Jonathan Barnes’ lab in the Department of Chemistry have developed a new light-sensitive hydrogel with improved biocompatibility compared with similar materials.

3-D printed, transplantable organs may sound like science fiction, but, thanks to advances in polymer chemistry, they could become a reality. Stimuli-responsive hydrogels represent a broad class of soft materials that change their mechanical properties when certain external triggers are applied. Last year researchers from the lab of Jonathan Barnes, assistant professor of chemistry, created a new kind of artificial molecular muscle from a polymer that changes color and contracts when exposed to blue light. Similar materials promise a wide range of applications, particularly in medicine.
Barnes started working on hydrogels because he wanted to develop a material that could change shape, size, and mechanical properties when actuated – just like our muscles do when they expand and contract. “A lot of people said we would never get the amount of contraction we were hoping for,” Barnes recalled, “but it actually worked better than we ever imagined.”
In “Reversible hydrogel photopatterning: Spatial and temporal control over gel mechanical properties using visible light photoredox catalysis,” published June 17 in the journal ACS Applied Materials & Interfaces, Barnes’ lab presented a new kind of responsive polymer that builds upon the success of the earlier study. The team’s new work focused on developing soft, biocompatible materials that could sustain heavy loads – materials that down the line may be particularly suited for medical uses like prosthetics or transplantable organs.
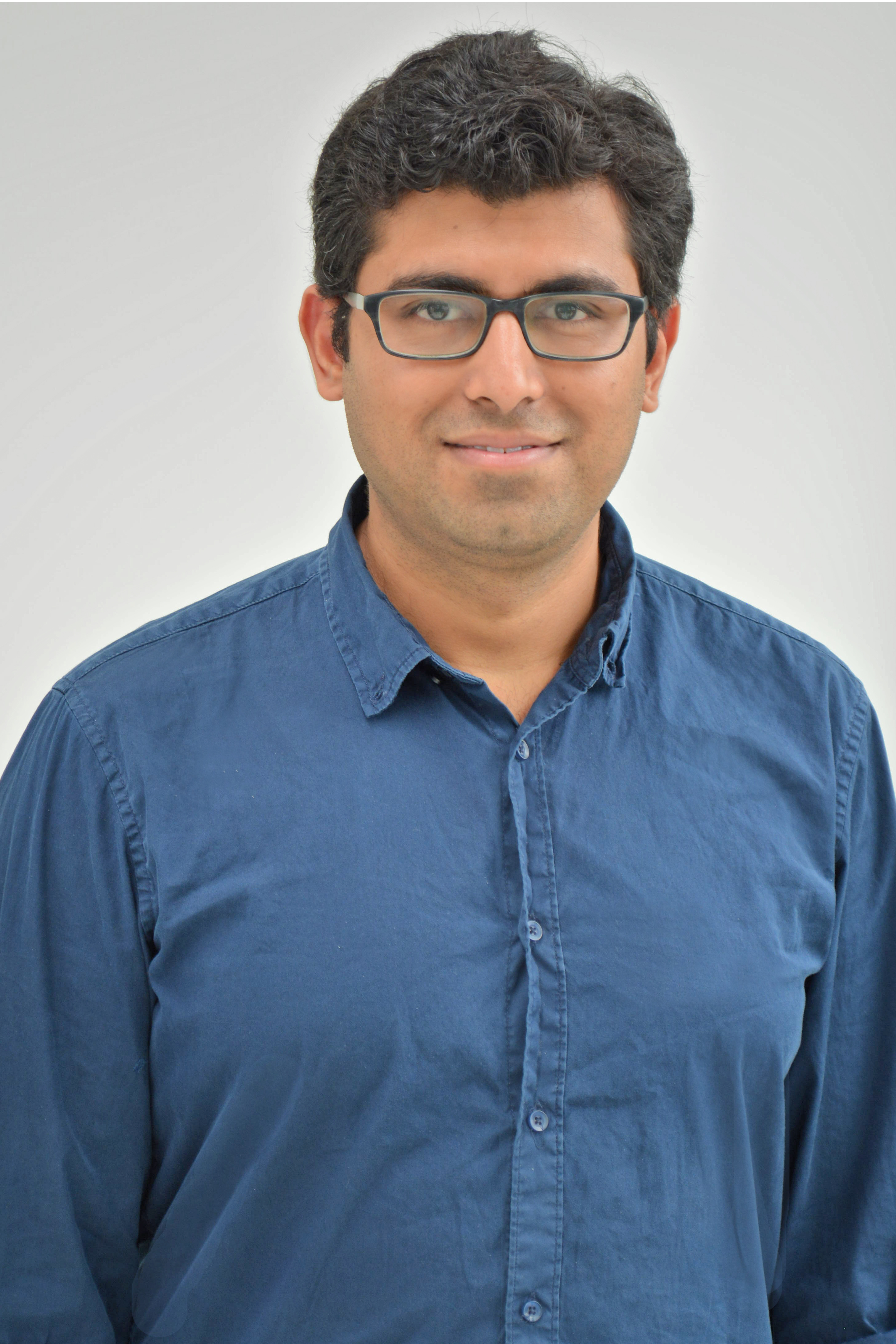
Faheem Amir, lead author on the paper and a postdoctoral researcher in Barnes’ lab, says that this type of study addresses a current gap in technology. “Cells within living systems face a 3-D environment, yet most of the studies that are done on cells are done on 2-D materials,” he explained. Hydrogels that are strong, yet soft and elastic, may provide a way for scientists like Amir to bring cells into a 3-D system and see how the cells behave under different conditions.
The team redesigned the chemistry behind their polymer’s reactions to create new hydrogels using a biocompatible polymer. The base material, currently used in contact lenses, allows for greater elasticity and can in turn better support 3-D cell networks.
Since their initial success, the team has worked to improve the speed of the reaction and its method of activation. Earlier hydrogels required submersion in a chemical reducing solution, but the team’s new material responds to visible light through the inclusion of a photocatalyst in the hydrogel network. This hot area of chemistry is known as photoredox catalysis, and it’s dramatically expanded the usability of Barnes lab’s hydrogels.
“We’re using the photocatalyst to absorb light and transfer an electron to our polymer, which actuates the material. As soon as we turn off the light and the material is exposed to oxygen in the ambient air, it reverses the process,” Barnes explained. “It’s kind of like a sponge. When we push all the water out, it’s smaller, but then when you drop it back in water, it will swell back up. It’s the same kind of process as in natural, mechanical systems, like muscles.”
Once the team knew the process would work using visible light, they wanted to refine their application by shining light on and activating only very precise locations in the gel, not all of it. That’s the focus of this study: Can the new hydrogel not only actuate as it did in previous iterations but also do so precisely?
Amir reported success in several areas. “The process resulted in significant increases in the soft material’s stiffness, tensile strength, and percent elongation before breaking, all of which could easily be reversed via oxidation and swelling in water,” he said. The hydrogels also allowed precise spatial resolution and control over where activations took place, which the team illustrated by photopatterning an American flag design.

Now that researchers in Barnes’ lab have spatial control over the activation of the hydrogel, they can turn to optimizing it for biomedical applications in collaboration with the Washington University School of Medicine (WUSM). “We know enough about the basic structure of organs that we should be able to 3-D print them in principle, but we’re lacking the materials,” Barnes said.
Researchers in Barnes’ lab will next focus on showing that their hydrogels are durable enough to support applications with cells suspended in a 3-D matrix. Being able to activate specific areas in three dimensions is a key step toward successfully growing tissue in a 3-D cell culture. Further refinements to the material will include activating it with other wavelengths of light, such as infrared, which would allow noninvasive activation through human tissue. The ultimate goal would be to create an injectable, 3-D-printable custom hydrogel -- a personalized “bio-ink” built from a patient’s own tissue -- that could be selectively activated through skin, just by shining light on it. This would allow for highly specialized applications within the body.
“Moving forward, we have developed a collaboration with Dr. Moe Mahjoub from WUSM where we are studying the effects of photoinduced actuation on cellular behavior,” Amir said. The collaborators hope their actuated hydrogels will be able to mimic human tissue, creating a general platform for use in countless applications. The versatility of the team’s key technology, their polymerizable cross-linker, supports this goal: Researchers can combine their cross-linker with any monomer to create custom polymers with carefully tuned features and mechanical properties.
“We took this idea that no one thought would work to the point where we’re actually showing biomedical relevance with these materials. This is pushing way beyond fundamental chemistry, and even beyond WashU, to build collaborations all around the country and even the world,” said Barnes. This research was presented at the American Chemical Society (ACS) meeting last April. Watch the full presentation by Barnes, “Muscle-like material expands and contracts in response to light,” from ACS Orlando 2019.