Chemists in Arts & Sciences are working to engineer proteins that may help counter devastating neurodegenerative diseases such as Alzheimer's, Parkinson's, and ALS.
The proteins in our bodies have evolved over many millions of years to reach their current diversity of functions, spurring on the necessary chemical reactions occurring within our cells, and protecting us from disease. Each has a unique three-dimensional structure to perform a specific function. But as our lifespans get longer, certain proteins become increasingly prone to take on alternative shapes from their evolutionarily stable ones. These misfolded proteins can ultimately trigger neurodegenerative diseases such as Alzheimer’s, Parkinson’s, and ALS. Meredith Jackrel, assistant professor in the Department of Chemistry, is developing strategies to make cells better able to deal with these tragic diseases. Jackrel and her research group are the recent recipients of a five-year grant from the National Institutes of Health and another from the Longer Life Foundation to study protein disaggregases, evolved protein forms that mitigate protein misfolding, as a strategy to combat these diseases. Her lab is exploring several naturally found protein disaggregases in hopes of engineering them to improve upon their capabilities.
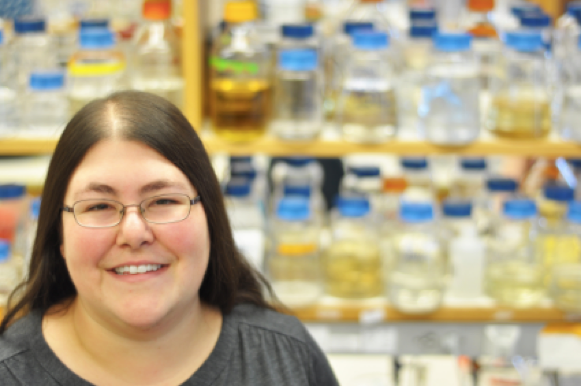
The building blocks that make up proteins are molecules known as amino acids. If proteins were sentences, amino acids would be the words that make them up and give them meaning. Fully formed proteins often naturally link up with other similar proteins in the body and fold together into distinct shapes. This folding process is fundamental to bodily function and continues throughout our life. However, it is possible for certain proteins to misfold, causing a malfunction that eventually, in the cases of proteins such as amyloid beta and tau, gives rise to Alzheimer’s disease. One of the focuses of Jackrel’s research group is studying the basic mechanisms associated with this misfolding and why it occurs at all. To do this, they use the tried-and-true experimental guinea pig, baker’s yeast.
A protein in yeast known as Hsp104 caught Jackrel’s attention during her postdoctoral studies. She and other researchers knew that yeast contained this protein to regulate prions, another word for misfolded proteins. They figured that if it could do this in yeast, then maybe it could work on the misfolded proteins that cause neurodegenerative diseases in humans. As a postdoc, Jackrel evolved Hsp104 to a form that did just that. “We figured out that Hsp104 can disassemble many different neurodegenerative disease-associated proteins. We also used protein engineering to develop a form of it that dissolves many different aggregated proteins such as TDP-43 in ALS and α-synuclein in Parkinson’s. Now the thought is that it can dissolve others,” said Jackrel. Since this protein is found in yeast, plants, and other microbes, but not animals, harnessing its potential for human use is a tall order, but researchers think there may be human proteins that could be employed in a similar way.
Maurie Balch, a postdoctoral research associate in the lab, studies chaperone proteins, the ones that normally protect proteins from misfolding. However, with aging, these proteins sometimes become unable to prevent misfolding. Thus she is testing HtrA1, a protein found in humans that may function similarly to Hsp104, to see if it can be engineered to prevent protein misfolding. “I hope within the next five months we are able to identify important features of HtrA1 responsible for activity against disease proteins as well as to understand more about the mechanism of HtrA1,” she said. Balch’s graduate work focused on how to control other chaperone proteins involved in warding off cancer, finding that certain small molecules allowed the chaperones to keep cancerous proteins at bay. After some time, she decided to transfer this knowledge towards studying neurodegenerative diseases. Added to Jackrel’s experience with protein engineering, this approach will, they hope, yield both practical results for potential drugs in the future and deeper understandings of protein misfolding, including how and why it occurs.
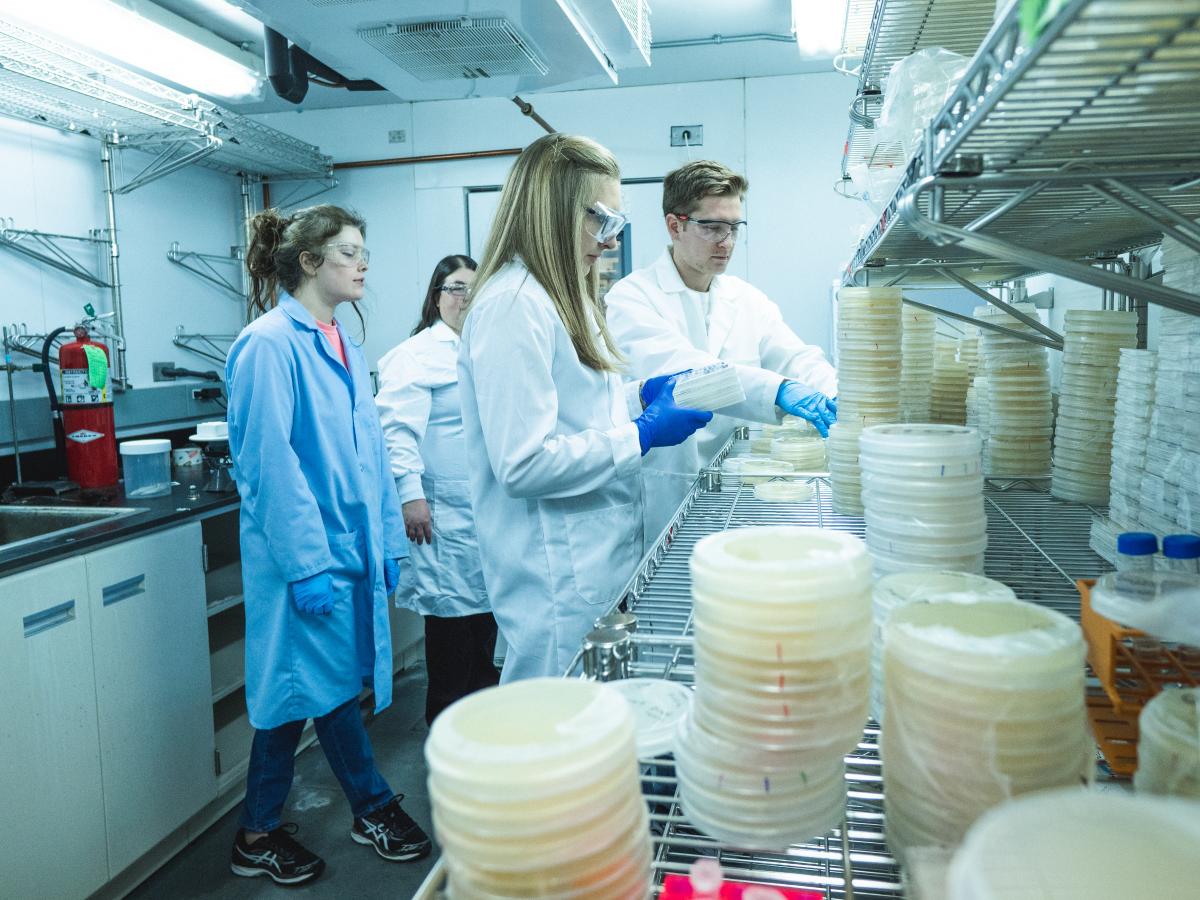
Jackrel’s focus is long-term – appropriately so given the intricacy and goals of her research. “There’s been major progress in the field,” she said. “I don’t think we’re quite there yet. The timeline for drug development is really long. Hopefully we’ll have something that’s better in a few years.”
The NIH grant that Jackrel received is part of a new initiative to fund more long-term research projects. Jackrel’s grant is for five years of research, giving the group much more stability and thus opportunity for more daring efforts. Much of her approach to protein engineering necessitates trial and error among many different protein sequences. The Hsp104 protein that alleviated the misfolding of multiple neurodegenerative disease proteins at once actually isn’t found in nature, rather it is a mutant arrived at through constant tweaking and engineering from its natural form. The research group will use similar engineering methods on HtrA1 to arrive, they hope, at a specialized form of its own.
Jackrel’s interdisciplinary work sits among a broad swath of projects based not only on the Danforth Campus, but also extending across Forest Park to the medical school. “I interact with a lot of people through the Hope Center and the Knight Alzheimer’s Disease Research Center, which has been great because I feel like I can be involved in so many research directions,” said Jackrel.
Just a year and a half into her career here at WashU, Jackrel and her team are hard at work in the lab with plans to publish promising results in the next few years – results that will, they hope, expand our knowledge of both the fundamental biochemistry of protein folding and possible approaches to countering these devastating diseases.