Physicists in Arts & Sciences will use X-ray emissions to gain insight into a supermassive black hole billions of light-years from Earth by using a distant galaxy as a magnifying glass.
Astronomer Carl Sagan once described Earth as a “mote of dust suspended in a sunbeam…a very small stage in a vast cosmic arena.” Comprehending the universe’s immense scale, in terms of both space and time, has long challenged our species. In disciplines like psychology and microbiology, scientists study complex systems using models that allow them to measure individual parameters through experiments and observations. But in the uncharted territories of deep space, novel observations are hard to come by and may require both cutting edge technology and the literal alignment of stars and galaxies. Few researchers understand these challenges better than doctoral student Andrew West and Henric Krawczynski, professor of physics and West’s research advisor.
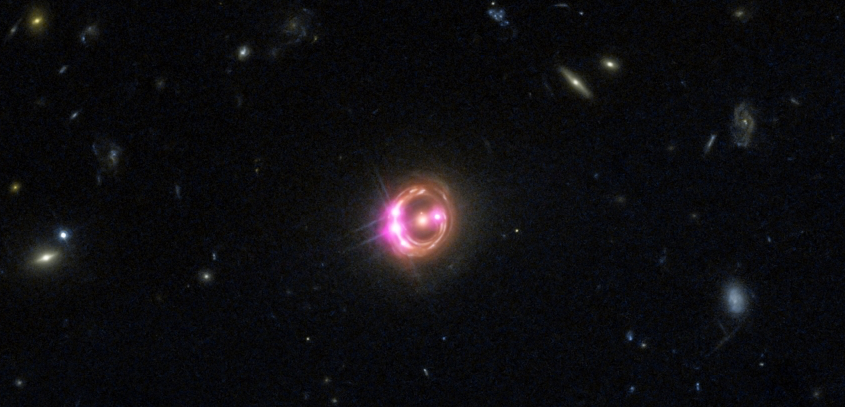
Krawczynski and West recently received an award from NASA to study RXJ-1131, a supermassive black hole located 6.05 billion light-years away from Earth. They will study the black hole using two satellite X-ray telescopes, Chandra and XMM-Newton, with orbits up to ten times greater than Earth's diameter. These telescopes detect X-rays from very near the black hole's event horizon – the surface that divides its "inside" from the "outside." Whereas matter and radiation can cross the event horizon from the outside to the inside, the reverse is not possible: Nothing that enters the black hole can ever escape.
"As the name implies, we can’t observe a black hole directly, but we can observe the material that’s accreting onto it,” said West. “As these materials move closer and closer to the black hole, they heat up and emit X-ray light. Ironically, black hole accretion disks are some of the brightest objects in the universe, and the energy of the light that they emit gives us information not only about the disk but also the black hole itself.”
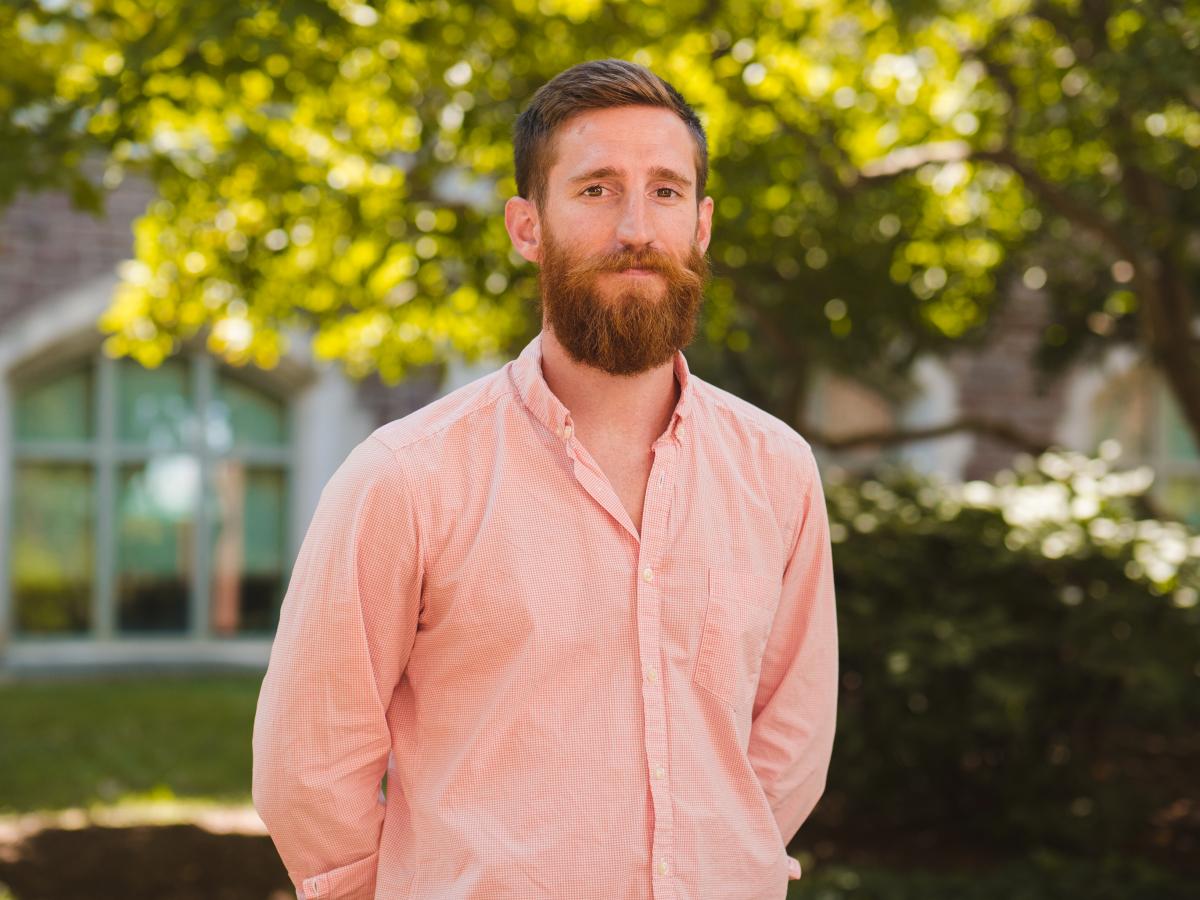
Chandra and XMM-Newton will be used to measure the intensity and energy of X-rays emitted by materials orbiting RXJ-1131. As this matter spirals toward the center of the black hole, it heats up to nearly one million degrees Kelvin (~1,800,000 °F) and emits X-rays that are characteristic of the material's composition. Since elemental iron is abundant and produces diagnostic X-ray energies, Krawczynski and West will primarily focus on energies associated with iron and investigate how these energies vary around the black hole. The research will be conducted in collaboration with George Chartas at the College of Charleston, who is the principal investigator of the NASA grant.
The iron atoms emit a very narrow range of X-ray energies, but due to the effects of gravity and the fast motion of gases near the black hole, the X-ray energies that are observed cover a much broader range. That range depends on how far the X-rays were emitted from the black hole and in which direction they were emitted. Like a police siren, an X-ray’s wavelength can be condensed or widened depending on whether it is moving toward or away from an observer – in this case, the satellites. The wavelengths experience additional widening as the X-rays fight to get away from the gravitational pull of the black hole. Krawczynski and West will measure how iron X-rays are shifted around RXJ-1131 and use these shifts to determine the spin of the black hole and tilt of orbiting materials. The observations will allow them to map the fabric of spacetime very near the black hole.
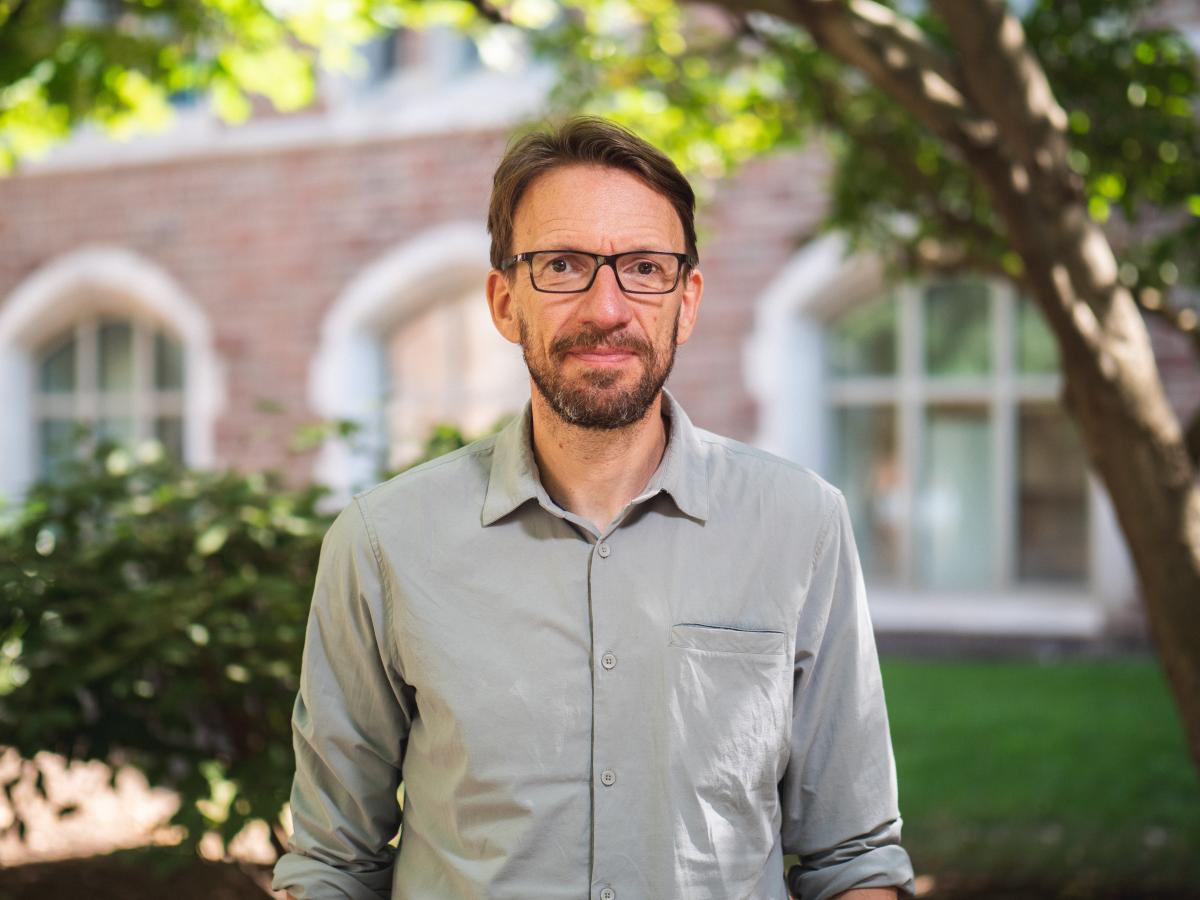
However, even with cutting-edge X-ray telescopes like Chandra and XMM-Newton, you need a strong signal to resolve these shifts in X-ray energies, which is particularly challenging to acquire when you're studying a distant black hole. Krawczynski and West will capitalize on the warping of spacetime to 'magnify' X-rays emitted around the black hole. In the astrophysical community, the magnification of an object through spacetime distortion is known as gravitational lensing. In principle, gravitational lensing is similar to a camera lens: Light is collected over a large area (the outermost lens on a camera) and directed toward a smaller area where a sensor records the incoming light.
In the case of RXJ-1131, the lensing galaxy is located approximately halfway between Earth and the black hole. The chance alignment of the lensing galaxy and RXJ-1131 to within a fraction of an arcsecond (1/3600th of a degree) across a significant portion of the observable universe makes this particular black hole a rare and interesting object for investigations with a wide array of telescopes.
As X-rays are emitted around the black hole, their intensities decrease with distance. Previous studies have used gravitational lensing to study distant bodies, but to more effectively overcome the decreasing intensities, Krawczynski and West will use a combination of gravitational lensing and microlensing to increase the X-ray flux further and improve the resolution of their measurements. Think of a microlens as a drop of water sitting on a pair of glasses: The droplet further magnifies the image behind the lens to reveal additional details. The less massive microlensing object, in this case a star within the galaxy, will help amplify a subset of X-rays emitted very close to the center of the black hole. In contrast, the primary (more massive) gravitational lens will amplify X-rays more weakly but from a much larger area. When both lenses are aligned, Chandra and XMM-Newton will receive a strong enough X-ray signal that Krawczynski and West will be able to resolve even more subtle shifts in X-ray energies.
West and Krawczynski will interpret the observations based on detailed computer simulations of the X-rays close to the event horizon of RXJ-1131 to account for the impact of the lensing galaxy and the microlensing stars on the received signal.
With such a complex lensing setup that relies on the literal alignment of a galaxy and star, it's virtually impossible to perform repeated, sequential experiments, which is why Krawczynski and West are commissioning two satellite telescopes. Together, these space telescopes will not only record a broader range of X-ray energies but also provide concurrent, overlapping measurements. By having two independent sets of X-ray measurements, Krawczynski and West will be able to test their methodologies more thoroughly and ultimately gather a more robust data set that can be used to evaluate existing models and theories.
Some might wonder why Krawczynski and West are so interested in a black hole that is 6 billion light-years away, and the answer is twofold: First, the universe is massive; in fact, the distance between Earth and RXJ-1311 is less than a tenth of the universe’s maximum width. But second, to understand our place in the universe, astronomers and astrophysicists must turn their gaze to stars as there is no analog to its vastness here on Earth.
Across disciplines, scientific models are used to study complex systems, but these models rely on more basic observations. For Krawczynski and West, observations are not made in a laboratory but rather in the depths of space and viewed through the lens of warped spacetime. Ultimately, Krawczynski and West aim to refine what the universe's parameters are and, in doing so, help us understand how we fit into it.
"Improving the accuracy of these measurements allows us to push the boundaries of human knowledge and test the veracity of fundamental theories,” said West. “Although the extreme conditions around a black hole might seem foreign to us here on Earth, they are prevalent throughout the universe. We need to understand these exotic objects in order to fully understand the universe and our place within it.”