Josh Blodgett, an assistant professor of biology, studies bacterial small molecule production. His research program uses interdisciplinary approaches to discover new bioactive molecules, understand how those molecules are made, and engineer microbes to increase their production. He sat down with the Ampersand to talk about how he integrates his industry background into his teaching and research.
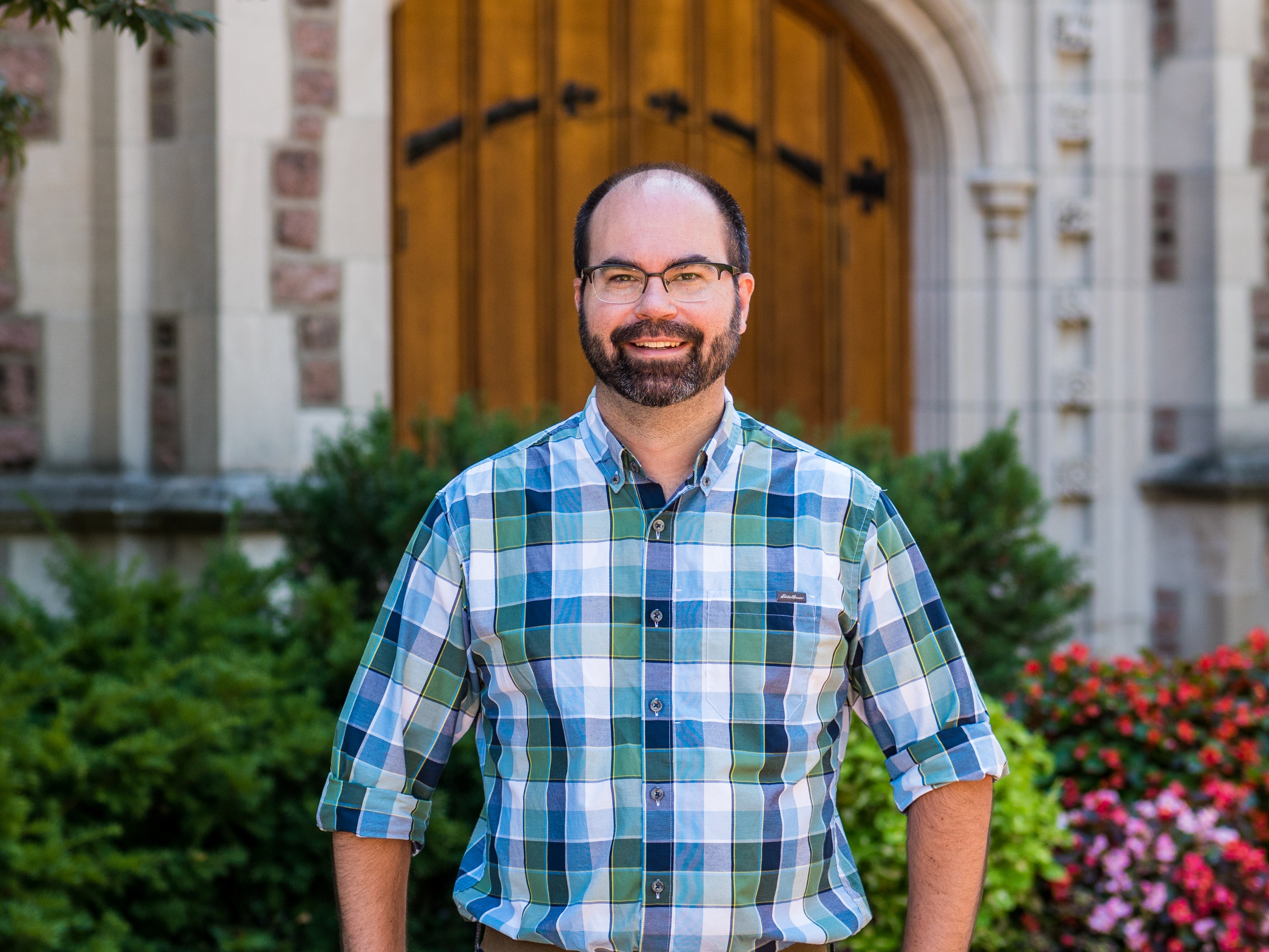
What does your lab work on?
We work on Streptomyces bacteria, microbes that make two-thirds of FDA approved anti-infectives. They are ubiquitous in the soil and freakishly endowed with the ability to produce drugs. We want to exploit these bacteria to make new potential drugs. We’re approaching this challenge in many ways. But to contrast our work with prior efforts in the field, we routinely leverage comparative genomics in our discovery work. And we want to understand how bacteria make drug-like compounds. Scientists have known about certain bacterially-produced drugs since the 70s, but we still don’t know how they are made!
How did you get interested in Streptomyces?
I loved microbiology as an undergraduate student. I got interested in it after taking a lab class; in one of the experiments, we plated soil dilutions on petri-dishes. On those plates, the microbes start to interact with each other. You could see the zones of inhibition, clearings around the Streptomyces, where they were inhibiting their neighbor’s growth. I thought it was remarkable.
Are there other microbes that make these kinds of compounds?
There are. But none produce the sheer number or the complexity (the chemical complexity) that comes from Streptomyces and related strains.
If scientists have known about these drugs for years, why do you think more compounds can be found?
Ten years ago, we knew that Streptomyces make 5-6 molecules per strain. But now, because of genomics, we know that many Streptomyces have 30-40 biosynthetic clusters. Each cluster forms a neat concise package of genes that direct the synthesis of these compounds. So, it looks like we have overlooked their capacity to produce these compounds significantly. Genomics has made it possible to discover previously missed drugs leads.
Given your industry background, what is the biggest challenge in drug discovery?
I come from an industry background (2.5 years in industry to help launch a pharmaceutical company) where we were using genomics to discover new drugs. If you want to go to market with different kinds of drugs, you need your bacteria to produce at least 1-10 grams per liter. These molecules are typically made at about ½ mg per liter; not very much. You have to industrialize the producers. Simply knowing the biosynthetic pathway (how the drug is made) won’t get you to 10 grams/liter. But what it can do is suggest simple steps that will take you to multiple milligrams per liter (50-100). That amount is often enough to start asking questions about the potential drug’s efficacy.
Have you discovered any new compounds?
Yes. One of my favorites comes from a Streptomyces strain I isolated from my grandparent’s garden in Connecticut, where I grew up. In 2010, I discovered that it makes a compound called clifednamide, which I named after my grandparents, Cliff and Edna. But unfortunately, this strain was a very poor producer of clifednamide, making it difficult to do experiments to learn about the compound’s activity.
How did you overcome that challenge?
We took multiple approaches. I got the undergraduates in my biotechnology course involved. I challenged them to go outside, grab a shovel full of dirt (the soil came from Tyson Research Center), and isolate new examples of these microorganisms. My BIOL 3493 students engage in series of experiments designed to teach the fundamentals of microbial drug discovery using genomics and traditional methods. This resulted in the isolation of strain KL33 named for Kevin Lou, the undergraduate who found it. Kevin’s strain turned out to be a better clifednamide producer than the original strain I’d found in Connecticut.
We also engineered a better producer. To do this, we figured out the clifednamide biosynthesis pathway – that is – deciphered how clifednamide is made. We learned that clifednamide is derived from another molecule, ikarugamycin, known to be effective against a wide-range of pathogens. We again turned to genomics and discovered a beautiful producer of ikarugamycin. Using our knowledge on how clifednamide is made, we inserted a single gene into the ikarugamycin-producing strain to transform it into a productive clifednamide producer. The engineered strain is the highest clifednamide producer discovered thus far.
Now that you can make a lot more clifednamide, what do you want to do next?
Ikarugamycin has activity in a broad array of organisms. It is an anti-ciliate, an anti-microbial, and a plant modulator. It has even been suggested to be anti-inflammatory. Now we have yet another analogue of ikarugamycin that we can create a lot more of. What I would love to do is to take this engineered microorganism or the compound it makes (clifednamides) and ask what happens when we put them in various plant models. Are ikarugamycin and clifednamides similarly effective? Do they help plants, or might they be involved in pathogenesis? You never know what you might find.